Using 4-D X-ray computer microtomography to observe high-temperature electrochemistry
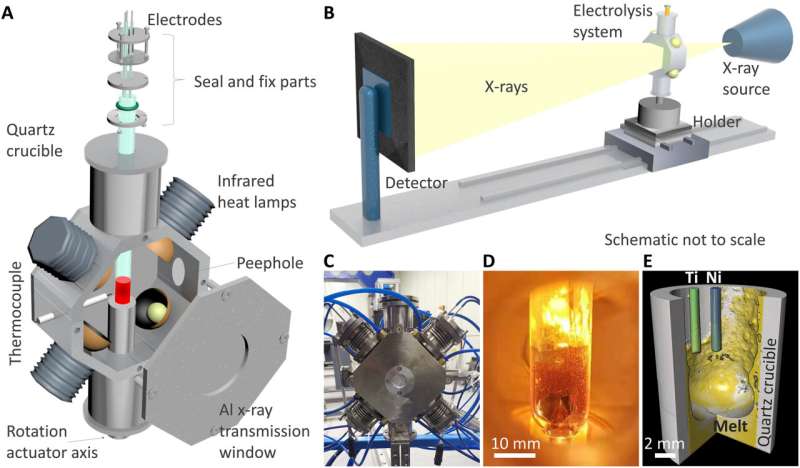
The concept of high-temperature electrochemistry has broad ranging applications in multiple fields; however, researchers yet remain to conduct real-time observations to gain in-depth understanding of the evolution in such systems. The primary limits include harsh reaction conditions and multiphysics fields. In a new report now published in Science Advances, Handong Jiao and a team of scientists in advanced structure technology and metallurgy in Beijing, China, addressed the challenge by developing a high-temperature electrolysis facility. The facility allowed in-situ X-ray computer microtomography (µCT) for non-destructive and quantitative three-dimensional (3D) imaging. The µCT further probed the dynamic evolution of 3D morphology and components of electrodes in 4D. The team visualized the 4D process using reconstructed images to monitor the efficiency of the process, and explore dynamic mechanisms to provide real-time optimization. The 4D analysis platform provided in-depth combinations of traditional electrochemistry with digital twin methods to extract data and facilitate multiscale visualization.
The experiments
High temperature electrochemistry has many applications across metallurgy, nuclear, chemical production and energy industries. The process can facilitate the molten salt/oxide electrolysis to extract and purify metal, with a prominent role in large-scale stationary energy storage transformation. The process of experimentally examining the evolution of internal dynamics in such systems remains challenging due to limited development of the method. To monitor the dynamic evolution under harsh temperature and electrochemical systems, Li et al. developed a specific high-temperature electrochemical facility with built in X-ray microtomography (µCT) for quantitative 3D imaging, including the morphology and components of electrodes within such systems under extreme conditions. The team verified the apparatus via classical electrorefining experiments of titanium in molten salt. They then performed a 4D study on the electrode structures and chemical components through time. The results combined high temperature electrochemistry with mathematical simulations to quantitatively design and optimize high-temperature electrochemistry.
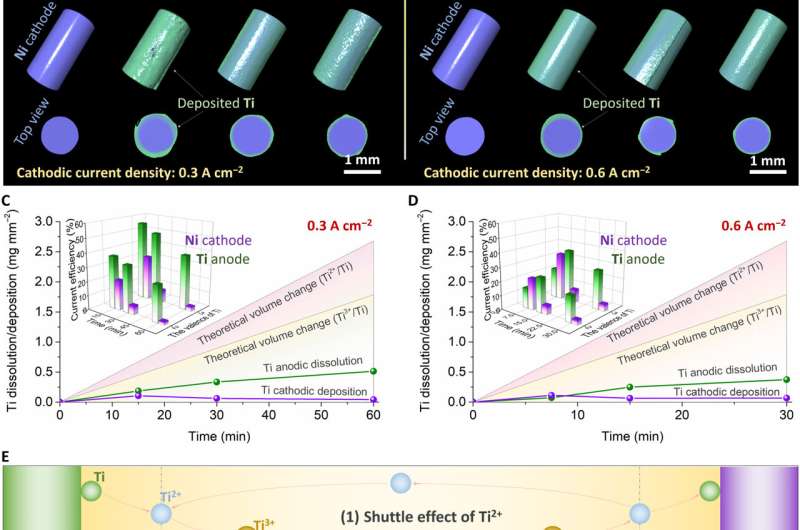
4D facility of high-temperature electrochemistry in the lab
The homemade in situ 4D characterization apparatus for high temperature electrochemistry contained a quartz tube electrolysis cell fixed vertically to the rotation actuator axis of the X-ray system. The team controlled the molten salt medium and electrodes by using an electrochemical measurement system based on metal wires. They then heated the cell by using four halogen lamps fixed to the heating furnace and settled the furnace chamber under vacuum conditions to reduce the temperature outside of the furnace. The researchers matched the distance between halogen lamps and cell to alter the heated zone according to experimental requirements. The electrolysis quartz cell provided an optically transparent view, although it was challenging to clearly observe the inner cell via an optical camera due to thermal radiation from high-temperature molten salt at elevated temperatures. The 4D facility based on X-ray µCT could reconstruct a reliable 3D complex structure of inner electrochemical cells with high spatial resolution from microscopic to macroscopic scales.
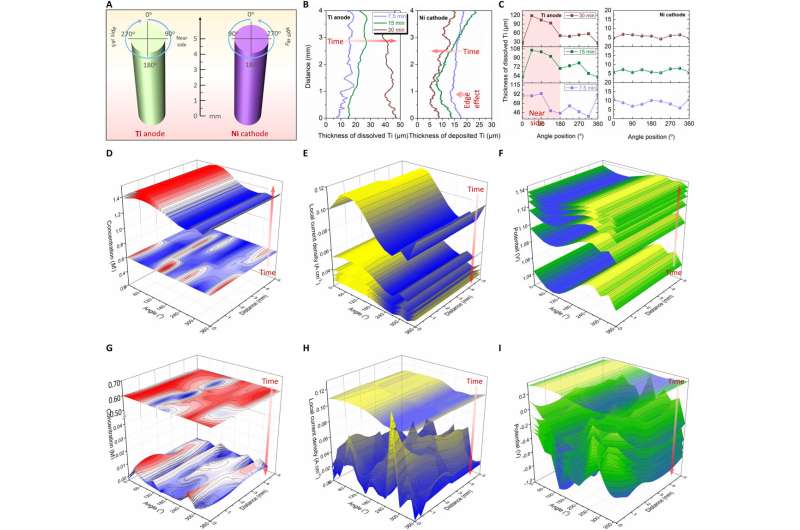
4D analysis
To show the capability of the 4D facility to conduct high-temperature electrochemistry, Jiao et al. performed a typical experiment of titanium (Ti) electrorefining in molten salt. The group had previously studied the extraction and purification of titanium using molten salt electroanalysis, with mechanisms of efficiency, purity and yield data yet to be understood. In this work, the scientists used a two-electrode system with titanium and nickel wires with same area and current density to form the anode and cathode. They then conducted scanning and electrolyzing in a cyclic process and obtained voltage-time profiles for the titanium electrorefining process under different current densities, then reconstructed 3D images of the Ti anode and Ni cathode at different electrolysis time ranges. Based on the outcome, Jiao et al. noted the average thickness of Ti plating on the Ni cathode to increase during the first electrolysis stage, to then drop slowly with time of electrolysis, which was unusual. The team therefore studied the competitive mechanism of chemical and electrochemical reaction at the interface and on the surface of electrodes to implement strategies to improve current efficiency.
Titanium dissolution and deposition
The scientists combined real-time experimental outcomes and simulations to reveal the evolution mechanism and extracted the local evolution information about the electrodes and noted the varying thickness of Ti anodic dissolution and Ti cathodic deposition surrounding the electrodes. The researchers noted the anodic dissolution and cathodic deposition of Ti to not be uniform. They revealed the evolution mechanism via multi-physical field simulations. Similarly, they obtained local information about the electrode surface to show the evolution of the 3D concentration field, current density and electric field surrounding the electrode along with electroanalysis time. Jiao et al. conducted additional experiments to understand the evolution of the 3D physical fields surrounding the Ni cathode. Based on the outcomes of the 4D characterization process, they optimized the electrorefining process in situ.
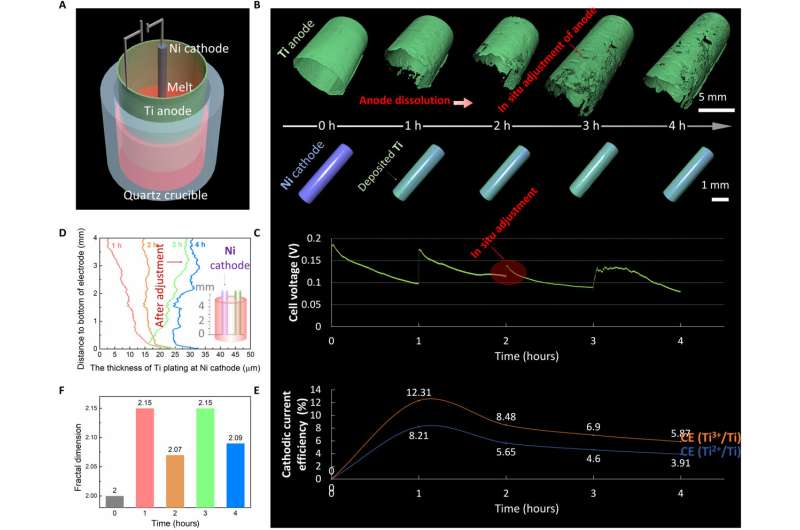
Outlook
In this way, Handong Jiao and colleagues successfully formed an in situ X-ray micro-computer-tomography method for high-temperature electrochemistry. The product offers new 4D characterization of the phenomenon for the first time. By using a titanium electrorefining experiment at high temperature, Jiao et al. verified the concept. They monitored the evolution of electrolysis for hitherto unknown quantitative details on electrode morphologies and current efficiency relative to different stages of electrolysis. The team combined the experimental results and multiphysics simulations to understand the mechanism of evolution in detail. The resulting techniques can be extended to high temperature electrochemical industries and industrial systems.
Scientists propose IAP process for separation of aluminum alloys
Handong Jiao et al, A 4D x-ray computer microtomography for high-temperature electrochemistry, Science Advances (2022). DOI: 10.1126/sciadv.abm5678. www.science.org/doi/10.1126/sciadv.abm5678
Angelique Ale et al, FMT-XCT: in vivo animal studies with hybrid fluorescence molecular tomography–X-ray computed tomography, Nature Methods (2012). DOI: 10.1038/nmeth.2014
© 2022 Science X Network
Citation:
Using 4-D X-ray computer microtomography to observe high-temperature electrochemistry (2022, February 21)
retrieved 21 February 2022
from https://phys.org/news/2022-02-d-x-ray-microtomography-high-temperature-electrochemistry.html
This document is subject to copyright. Apart from any fair dealing for the purpose of private study or research, no
part may be reproduced without the written permission. The content is provided for information purposes only.
For all the latest Science News Click Here
For the latest news and updates, follow us on Google News.