The evolutionary role of actin within sophisticated architectures of biosilica in sponges
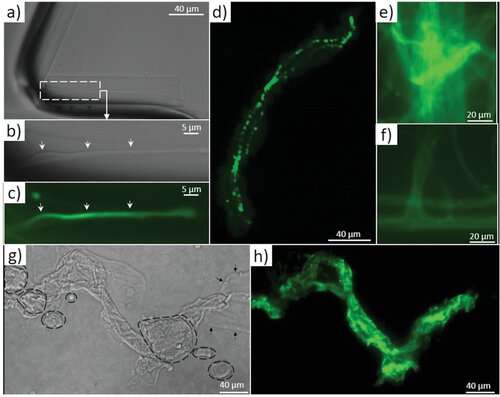
Spiculogenesis or the growth of glass-like structures and biomineralization in early sponge species have led to the realization of highly regular glass architectures in nature. In a new report now published in Advanced Science, Hermann Ehrlich and a team of international scientists investigated a previously unknown role of the protein actin.
The team of biomineralogists used modern bioanalytical methods to show the presence of the protein actin in glass structures of sponges to ask a key question: “how does biological glass grow?” Actin is a fundamental member of an ancient superfamily of structural intracellular proteins and plays an important role in the cell cytoskeleton and in cellular dynamics in both prokaryotes and eukaryotes. The protein has additional functions in metazoans, patterning the deposition of biosilica for more than 500 million years. In this work, the team provided an answer to a fundamental question in bioinspired biomaterials science relative to the growth of ‘biological glass’ to show how axial filaments are patterned in the silica architecture of metazoans in vivo. The scientists also showed why metazoans grew past ambient temperatures (from -1.9°C to 24°C) to reach dimensions in the meter range.
Actin is full of surprises
Lead author of the study, Hermann Ehrlich, who is professor and head of the Biomineralogy and Extreme Biomimetics research group at the Freiberg University of Mining and Technology in Germany, commented on the process as “one of the most thoroughly studied fundamental proteins that still holds surprises. … recent findings showed how actin played a new role to organize the pattern of the skeleton to the most basal of animals—the sponges.” The researchers summarized their strategy as “an attempt to see what everyone has seen, and to think what nobody has thought.” To accomplish this ambitious research plan relative to the role of actin in marine sponges, Ehrlich et al. employed a range of bioanalytical methods, including proteomics, Western blotting, phalloidin staining, immunostaining, high-resolution transmission electron microscopy, Raman spectroscopy and Fast Fourier transform techniques. Using these methods, the team showed how axial filaments in a range of representative sponge classes, including Hexactinellida and Demospongiae were made of F-actin. Ehrlich said, “Amazingly, even giant axial filaments of the giant glass sponge Monorhaphis chuni are composed of F-actin, to reveal the largest actin filament bundles ever reported.”
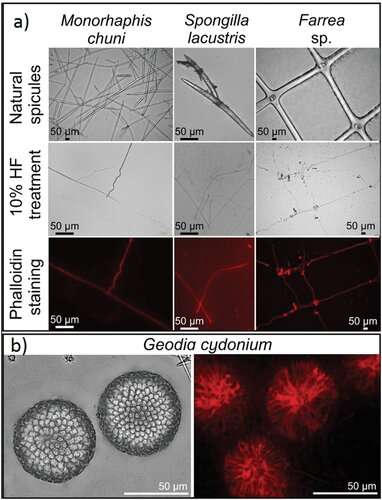
An ancient role with a modern twist.
The team highlighted how the conserved morphology of sponge biomaterials describe an ancient role that F-actin had played for at least 545 million years. In the study, Ehrlich et al. proposed F-actin to be localized in an ancestral intracellular siliceous construct space. The growth of glass-like structural particles known as spicules or spiculogenesis could have moved the materials to extracellular spaces, where the actin continued to play a pattern-forming role. The scientists showed how actin within such glass structures appeared to perform a new function as a driving force to define the diversity of sophisticated biosilica structures in sponges. During the study, the researchers developed a new method to work at the microscopic scale, to isolate axial filaments from siliceous microstructures derived from diverse species of sponges. The scientists gently applied a drop of 10 percent hydrofluoric acid onto the surface of a spicule, to facilitate the acid to dissolve the biosilica and leave the organic matrix intact. Using the approach and accompanying immunofluorescence staining, Ehrlich et al. identified actin in the axial filaments of a variety of sponges.
-
Identification of actin in the axial filament of the glass sponge Asconema setubalense. a) iFluor 594-Phalloidin red staining of partially demineralized spicules shows the axial filament. b) The amino acid sequence of actin (UniProt ID A0A1Y9T597) was identified by the MS proteomic approach in the axial filament of A. setubalense. Seven peptides were identified using an in-solution digestion and label-free nanoLC-MS/MS approach (marked in bold), yielding 27.1% coverage of a protein sequence (Table S1, Supporting Information). c) SDS-PAGE analysis of the A. setubalense actin filaments indicating the presence of 42 kDa band visualized by Coomassie blue G-250 staining (1# and 2# marked by an asterisk). Lanes #1 and #2 correspond to axial filaments isolated from two different specimens of the same sponge. Four actin peptides were found in this band by MS protein identification (bold and underlined peptides), with 13% sequence coverage. d) Western blot analysis with a human anti-ß-actin antibody confirmed the actin signal at 42 kDa (marked by an asterisk). Credit: Advanced Science (2022). DOI: 10.1002/advs.202105059
-
TEM imagery and Fourier analysis of the axial filaments isolated from spicules of the glass sponge M. chuni. b) Zoomed image of a) the TEM image that represents nanofibrillar organization of the selected area of isolated axial filament of M. chuni shows the cross-linked nanoarchitecture (arrows) typical for F-actin filaments, which form right-handed, parallel, and staggered structures in all eukaryotes.[38, 41] The FFT in (e) taken from (d) indicates different large periodicities typical for actin such as 5.9, 4.6, 3.7, 3.4, and 1.9 nm. Individual reflections are shown with red colored dotted lines. d) The axial filament lattice shows high similarity to that of actin standards reported by other authors. Credit: Advanced Science (2022). DOI: 10.1002/advs.202105059
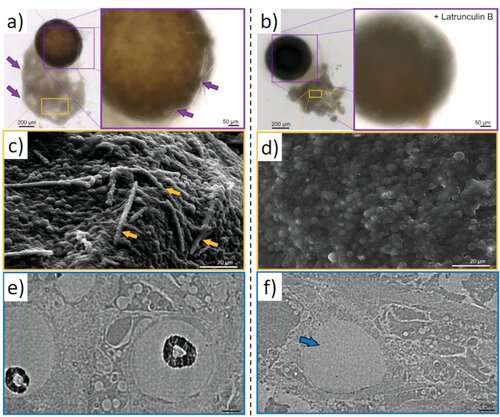
Characterizing the actin filaments
The research team used a wide array of experiments—for example, by using proteomics—to reveal actin as a component of axial filaments, while also revealing the presence of actin in spicules of glass sponges. They also used high-resolution transmission electron microscopy to view and understand the periodic structure of actin, followed by Fourier transform imaging to determine the structure of these crystals, while investigating nanostructural organization of axial filaments, typical of F-actin. They then conducted inhibition assays to prevent spicule formation to explore the role of actin during the development of spicules in vivo. The outcomes of the research echoed previous work, which showed how the disruption of the actin-network interfered with the secretion of biomineralized elements in marine unicellular haptophytes. Ehrlich et al. envision a bold direction for the research outcomes, writing that these findings “will have an impact on research in a diverse range of disciplines, including bioinspired materials science, biomechanics, biomineralization, the chemistry of science, functional materials, and biomimetics.”
Outlook for extreme biomimetics
The team intend to conduct in vitro polymerization of actin monomers into filaments under model conditions in the presence of silicic acid. In this way, Hermann Ehrlich and colleagues confirmed the presence of actin in silicified skeletal constructs of select sponges from cold and warm water. The wide temperature range confirmed the presence of actin as actin-rich axial filaments in Hexactinellida and Demospongiae sponges to generate patterns and create the amazing structural diversity of biosilica-based skeletons—a source of inspiration in biological materials science. The team assumed the role of actin to be ancient due to morphological conservation of sponges through fossil records for at least 545 million years. They proposed the evolution of F-actin in ancestral intracellular constructs with the intention of creating sophisticated 3D biomaterials and new silicate-containing materials in the lab. The scientists write, “The prospect of creating new silicate-containing materials by using actin filaments to maintain three-dimensional architectures will be extremely interesting to form a biomimetic model and examine in-lab mineralization.”
Hermann Ehrlich et al, Arrested in Glass: Actin within Sophisticated Architectures of Biosilica in Sponges, Advanced Science (2022). DOI: 10.1002/advs.202105059
Hermann Ehrlich et al, Mineralization of the metre-long biosilica structures of glass sponges is templated on hydroxylated collagen, Nature Chemistry (2010). DOI: 10.1038/nchem.899
© 2022 Science X Network
Citation:
The evolutionary role of actin within sophisticated architectures of biosilica in sponges (2022, April 8)
retrieved 8 April 2022
from https://phys.org/news/2022-04-evolutionary-role-actin-sophisticated-architectures.html
This document is subject to copyright. Apart from any fair dealing for the purpose of private study or research, no
part may be reproduced without the written permission. The content is provided for information purposes only.
For all the latest Science News Click Here
For the latest news and updates, follow us on Google News.