Study shows shaker channel mutation differs structurally from human potassium channels
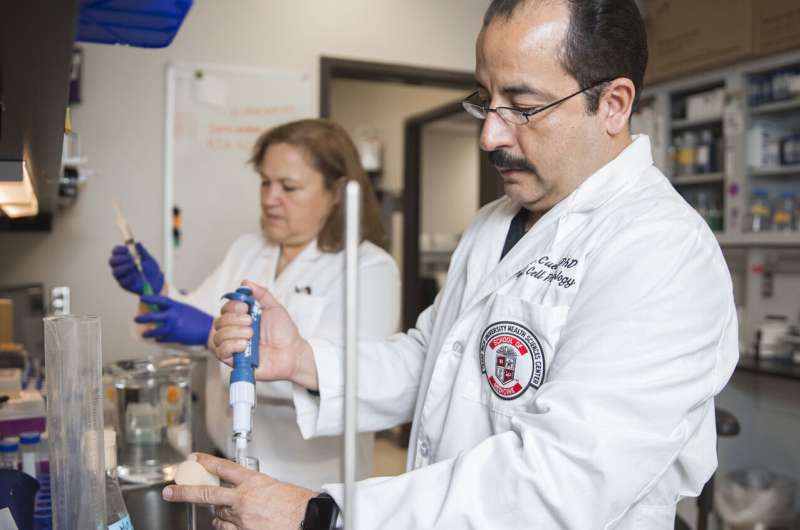
Potassium (K+) channels are small, highly specialized conduits within every living cell that are responsible for the extremely selective and rapid transport of K+ ions across cell membranes. Voltage-gated potassium (Kv) channels are potassium-specific transmembrane channels that also are sensitive to voltage changes within the cell’s membrane where a selectivity filter chooses K+ ions over sodium (Na+).
In addition to directing ion selectivity, the selectivity filter employs a process known as C-type inactivation, which allows the selectivity filter to act as an additional gate that can stop the flow of ions. C-type inactivation is a rearrangement of the selectivity filter that occurs when prolonged depolarization of the cell membrane is triggered by opening the membrane’s activation gate.
In a study led by Luis Cuello, Ph.D., from the Texas Tech University Health Sciences Center (TTUHSC) School of Medicine, and Alain J. Labro, Ph.D., from the University of Antwerp and Ghent University in Belgium, the researchers investigated whether or not a known mutation (W434F) found within Shaker-IR (inactivation removed) channel epitomizes and functionally accelerates the C-type inactivation state.
The Cuello-Labro team included D. Marien Cortes from TTUHSC and Laura Coonen, Evelyn Martinez-Morales, Dieter V. Van De Sande and Dirk J. Snyders from the University of Antwerp. Their study, “The nonconducting W434F mutant adopts upon membrane depolarization an inactivated-like state that differs from wild-type Shaker-IR potassium channels,” was published in September by Science Advances.
The unique transport mechanism of K+ ions define the correct functional behavior of every living cell. In doing so, the K+transport mechanism effectively controls a variety of very complex processes such as the normal electrical activity of brain neurons, the human body’s typical immune response against life threatening pathogens and the rhythmical beating of the human heart.
Within the human heart, one of these potassium channels, the hERG channel, must undergo C-type inactivation before it can function as necessary to maintain the heart’s periodicity, or the time interval between beats. Cuello said these potassium channel proteins are in a resting state in a normal cell, but must be activated to work properly. However, for the normal functioning of the human heart, it is crucial that they undergo C-type inactivation.
“After it does activate, the channel has to undergo inactivation,” Cuello explained. “That is so important for the human heart because it needs to keep its periodicity, and the heart beating is based on that feature; the channel has to inactivate.”
To learn more about the Kv channels in humans, scientists have for many years studied a specific mutation (W434F) within the Shaker channel, a potassium channel derived from the Drosophilia melanogaster, a common species of fruit fly. Similar to the potassium channels in the human body, the Shaker channel is comprised of vital membrane proteins that play an essential role in the proper functioning of the cell and have a role in potassium ion channel operations.
The Shaker channel was discovered in the U.S. about 50 years ago and was made widely available, especially to those studying potassium channels, because it was the only channel available. When a mutation was discovered on the channel—W434F—it was widely accepted to represent the C-type inactivated state of a potassium channel. Cuello said his team has shown that is not the case.
“In this paper, we prove that the normal channel that inactivates has a structure and a conformation which is different from that of the mutant,” Cuello said. “Thanks to our work, we said, ‘Hey, be cautious because that mutant channel is a very different conformation, or you might have a different structure which doesn’t have anything to do with the real channel in the heart or in the human body.’ This is important because if you want to design a drug based on this assumption that the normal channel looks like the mutant channel, that’s going to be incorrect because it seems to be that in the inactive state, the structure of the W434F is different based on our experimental result.”
The mutant channel has been used because it basically produces a channel that doesn’t pass ions. Cuello said there were some experiments that suggested the mutant doesn’t conduct ions because it is inactivated like normal potassium channels in the human body. However, the results from his team’s experimental studies strongly suggest the mutant channel is trapped in a deep inactivated and non-physiologically relevant conformation. And because the human potassium channels are very similar to the mutant, the Shaker channel has been considered for years to be a structural surrogate to study all the channels.
“That particular mutant (W434F) is not a good comparison to the C-type inactivated human channel that we have in the body, so we have to be cautious with it,” Cuello stressed.
It also was believed that the channel lacks the selectivity of potassium or sodium, but Cuello said his team’s work proved that isn’t the case.
“The channel still remains potassium selective; the only thing that it doesn’t do is conduct potassium.,” Cuello said. “That’s important when you want to design new drugs because that provides you information about which part of the protein you must tap to tackle it as a therapeutic target. In this case, we are calling attention to our work and saying, ‘Hey, do not establish a structural parallel between the mutant inactivated channel and the inactivation state of a normal channel in the human body.’ Be aware there is no structural equivalent, and that’s extremely important.”
As his research moves forward, Cuello’s lab is moving away from studying the fruit fly K+ channel and has begun working with three isoforms of human K+ channels. Two of the channels they are working with are expressed in the immune system, and one channel is expressed in the brain.
“We want to study what happens when these channels inactivate,” Cuello reasoned. “How is that process here, and how we can design specific drugs to tackle a specific state of the channel? Do we need to target the channel when it is in the closed state, or do we need to tackle the channel when it’s in the open state? We need to start studying the human isoform of all the potassium channels if we want to design specific drugs for specific diseases.”
Research team reverse way potassium channels work from bacteria to human
Laura Coonen et al, The nonconducting W434F mutant adopts upon membrane depolarization an inactivated-like state that differs from wild-type Shaker-IR potassium channels, Science Advances (2022). DOI: 10.1126/sciadv.abn1731
Citation:
Study shows shaker channel mutation differs structurally from human potassium channels (2022, October 12)
retrieved 12 October 2022
from https://phys.org/news/2022-10-shaker-channel-mutation-differs-human.html
This document is subject to copyright. Apart from any fair dealing for the purpose of private study or research, no
part may be reproduced without the written permission. The content is provided for information purposes only.
For all the latest Science News Click Here
For the latest news and updates, follow us on Google News.