Study demonstrates the robust storage of qubits in ultracold polar molecules
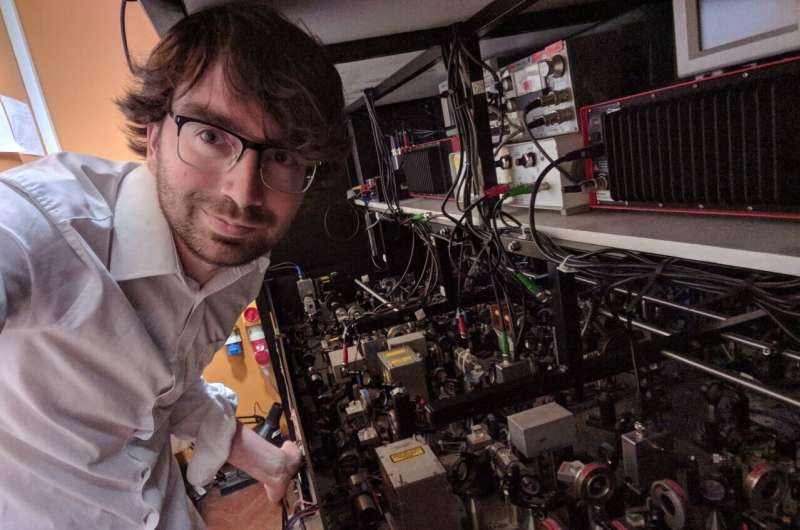
Molecules have a very intricate and rich structure, which allows them to rotate and vibrate freely. As a result, they have an almost limitless space in which computer scientists could encode quantum information. In addition to their vast internal space, molecules are capable of long-range interactions and could thus be entangled to other separate molecules.
Due to these advantageous qualities, many engineers and physicists have been investigating the potential use of molecules for quantum computing applications. While some quantum computers based on molecules have achieved promising results, scientists have found that qubits stored in molecules are susceptible to decoherence (i.e., a loss of information that travels from a quantum system to its surrounding environment).
Researchers at Durham University in the U.K. have recently carried out a study aimed at investigating the potential of storing quantum information in ultracold polar molecules. In their paper, published in Nature Physics, the team successfully demonstrated the storage of qubits in molecules while limiting decoherence, which could have important implications for the development of quantum computing tools.
“One of the great challenges facing all quantum computation platforms is developing engineering methods that avoid losing quantum information through decoherence,” Philip D. Gregory and Simon L. Cornish, two of the researchers who carried out the study, told Phys.org via email. “Our primary objective was therefore to demonstrate that quantum information could be stored in a molecule for exceptionally long times, thereby addressing one of the requirements for building a quantum computer using ultracold molecules.”
The primary objective of the recent work by Gregory, Cornish and their colleagues was to identify, understand and ultimately eliminate all experimentally relevant sources of decoherence in molecule-stored qubits. Initially, the team measured coherence in their quantum system using a technique known as Ramsey interferometry at different states of the qubit. They then prepared a superposition of the qubit states using microwaves and allowed the system to evolve over time.
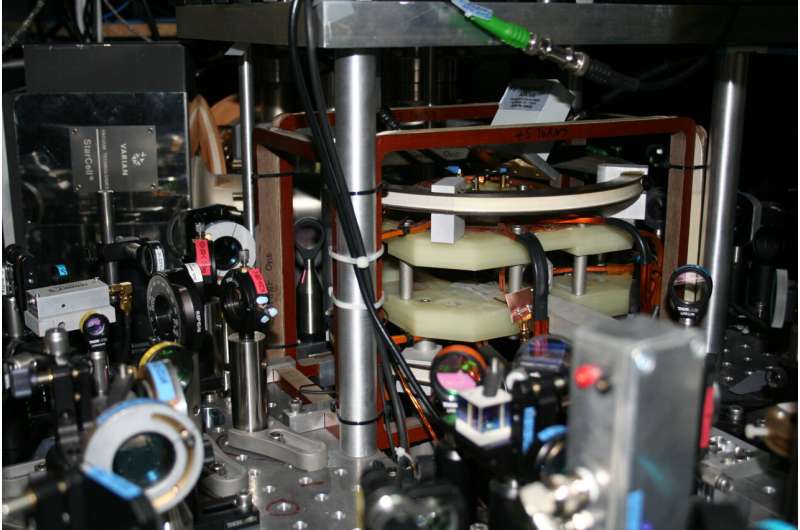
“To test the coherence, we used a second microwave pulse that leads to phase-dependent interference between the state populations,” Gregory and Cornish explained. “What we observed are oscillations in the number of molecules in either state of the qubit as a function of time, and we also found that decoherence is characterized by a reduction in the amplitude or contrast of those oscillations.”
Using their microwave pulses-based method, Gregory, Cornish and their colleagues were able to examine the coherence time as a function of any parameter used in their experiment (e.g., the magnetic field or the polarization of the trapping light), simply by changing the value of the parameter for the period between the microwave pulses in the Ramsey sequence. Finally, they compared their findings to a detailed model of the rotational and hyperfine structure of the molecule the qubits were stored in. This allowed them to understand the unique roles of different interactions within the molecule that may contribute to the system’s loss of coherence.
“Our most important achievement is the elimination of decoherence sources in our experiment,” Gregory and Cornish said. “This has implications for quantum computing with ultracold molecules, as quantum information can now be stored for much longer periods of time.”
In their experiment, the researchers were able to eliminate sensitivity to magnetic field noise by identifying a pair of hyperfine states that, when subjected to a specific magnetic field, have an energy difference between them that does not depend on small changes in the magnetic field. In addition, Gregory, Cornish and their colleagues uncovered a subtle tensor light shift between the qubit states. Nonetheless, they showed that this shift could also be eliminated by carefully choosing the polarization angle of the trapping laser.
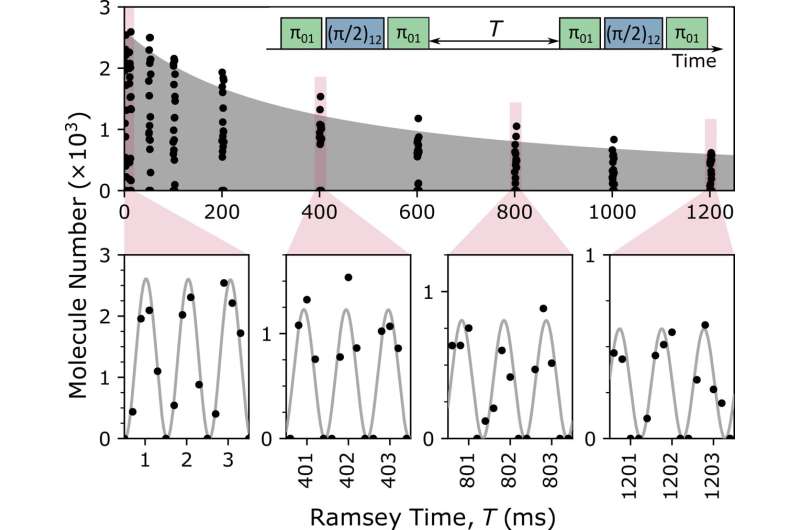
“Remarkably, having eliminated all these sources of decoherence, we found that the coherence time was much longer than the lifetime of our molecular gas (which is limited by collisional loss),” Gregory and Cornish said.
In the future, this work could inform the development of new quantum technologies that store information inside molecules. In addition, it could have valuable implications for the collection of measurements, as the techniques used by Gregory, Cornish and their colleagues enables particularly long interaction times with molecules. This means that it could be used to collect highly precise measurements at hyperfine states of molecules, which could in turn broaden the current understanding of their internal structure.
“Gate operations with ultracold molecules are possible using resonant dipole-dipole interactions,” Gregory and Cornish said. “Such interactions may be accessed using the rotational states of the molecule. We are currently developing a rotationally magic trap, where the light shift of the ground and first rotationally excited states will be identical. Such a trap will support long coherence between rotational states, which will be important for implementing high fidelity entangling gates, as well as studying models relevant to quantum magnetism.”
For several quantum computing applications, the use of ultracold molecules is only possible if molecules are confined within a controllable spatial array and can be individually monitored and accessed. Gregory, Cornish and their colleagues are thus now also working on a strategy for loading molecules into optical lattices and assembling individual molecules in arrays, storing them within optical tweezer traps.
“Isolating the molecules in this way will also prevent collisions between molecules,” Gregory and Cornish added. “This will increase the available interaction time further and will allow us to put better limits on the coherence time in the future.”
Shielding ultracold molecules with microwaves
Philip D. Gregory et al, Robust storage qubits in ultracold polar molecules, Nature Physics (2021). DOI: 10.1038/s41567-021-01328-7
© 2021 Science X Network
Citation:
Study demonstrates the robust storage of qubits in ultracold polar molecules (2021, September 27)
retrieved 27 September 2021
from https://phys.org/news/2021-09-robust-storage-qubits-ultracold-polar.html
This document is subject to copyright. Apart from any fair dealing for the purpose of private study or research, no
part may be reproduced without the written permission. The content is provided for information purposes only.
For all the latest Science News Click Here
For the latest news and updates, follow us on Google News.