A versatile hydrogel network-repairing strategy
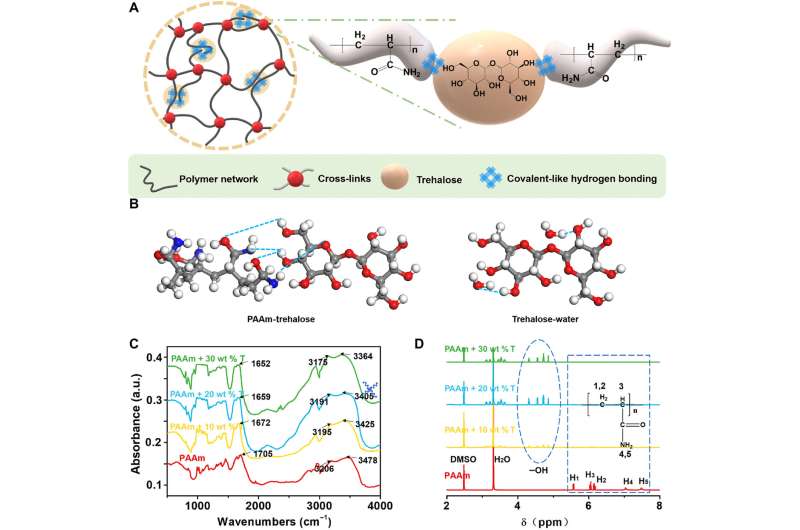
Hydrogen bond engineering can convey stretchability, toughness and self-healing properties to materials, although enhancement effects of conventional hydrogen bonds is limiting due to their weak interaction strength. For instance, organisms can withstand extreme conditions due to strong hydrogen bond-based interactions induced by trehalose. In a new report now published in Science Advances, Zilong Han and a team of scientists in smart devices and engineering mechanics at the Zhejiang University in China, described a trehalose network repairing method. They achieved this based on covalent-like hydrogen bonding interactions to improve mechanical properties of hydrogels, to simultaneously allow them to tolerate extreme conditions while maintaining synthetic simplicity useful for a variety of applications. The trehalose-modified hydrogels offered multiple mechanical properties, including strength, stretchability and fracture toughness under a range of temperatures. After dehydration, the team noted the maintenance of hyperelasticity and retained the functionality of modified materials compared to unmodified materials. The method is a versatile process to synthesize well tolerant and highly stretchable tough hydrogels for broad applications.
Hydrogel networks and bioinspired advances
Hydrogels are biocompatible, conductive and mechanically tunable materials with optical transparency, ideal for tissue engineering, micro-lens development, as ionic conductors and for soft robotics. Many applications rely on the structure, stability and mechanical properties of materials under a variety of conditions, including dehydration and low temperatures. Nevertheless, network imperfections in hydrogels have considerable effects on mechanical properties leading to their degradation, which can be exacerbated due to water crystallization at low temperatures; resulting in the malfunction of such material-incorporated devices. Due to the existing gap between theory and experiments, there exists much potential in materials science to repair hydrogel network imperfections. Materials scientists are often inspired by nature to develop bioinspired materials. For instance, water bears (tardigrades) can tolerate extreme temperatures to withstand almost complete dehydration and high pressures to which they adapt by synthesizing large amounts of trehalose. Trehalose molecules can stabilize biomolecules via strong interactions between hydroxide groups in trehalose and polar groups in biomolecules, while protecting against water crystallization at low temperatures. Trehalose and its derivatives are environmentally biocompatible and benign, Han et al. were therefore inspired by their unique properties to form an effective repairing agent for hydrogels, based on covalent-hydrogen bonding interactions, to impart self-healing properties.
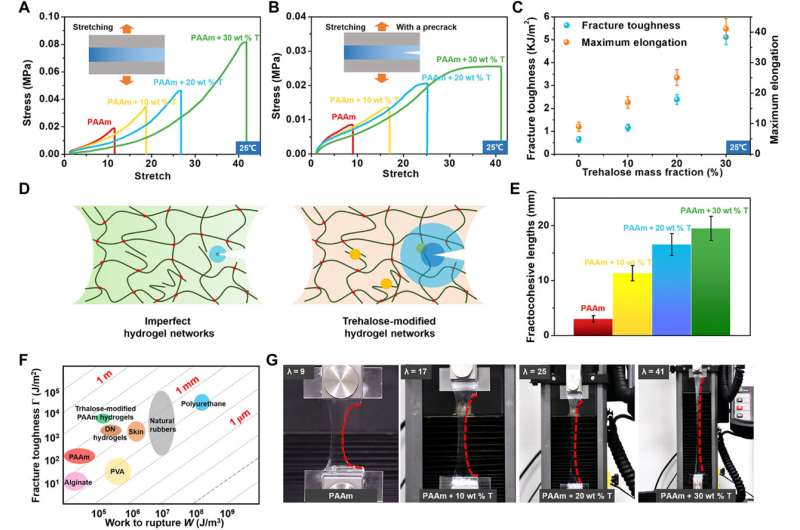
Trehalose network-repairing method
Hydrogel networks maintain a variety of imperfections including chain and loop defects. Han et al. showed how the addition of trehalose into polyacrylamide (PAAm) hydrogels led to the formation of strong hydrogen bonds between trehalose molecules and polar groups within long polymer chains. Many pathways of interaction exist between trehalose and the hydrogel network. The research team used the “Materials Studio’ to study the interaction strengths among water molecules (W-W), trehalose (T-W), and PAAm molecules (P-W) in the hydrogel, and simulate the molecular structures to calculate interaction energies based on density functional theory (DFT). The outcomes showed the interaction energy between trehalose-water (T-W) to be smaller compared to those between water-water and polymer-water. On the basis of DFT calculations, Han et al. illustrated the P-T (polymer-trehalose combination) to indicate quadrupole hydrogen bonds with an interaction energy of -68.36 Kcal/mol—of a strongly covalent nature.
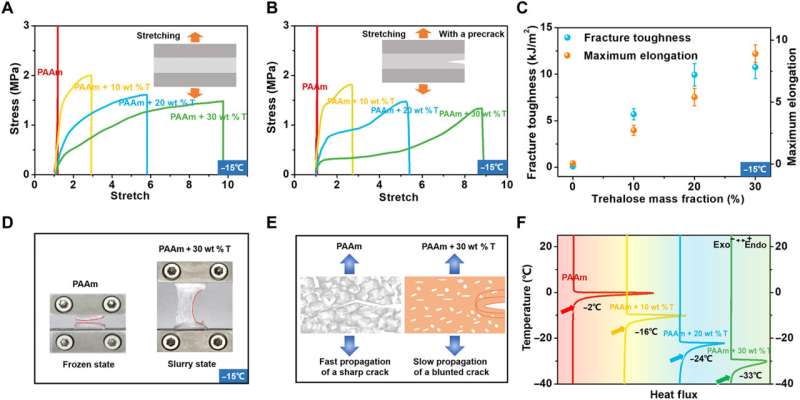
Characterization study and enhancement effects
The research team used Fourier transform infrared (FTIR) spectroscopy and proton nuclear magnetic resonance (1H-NMR) spectra to characterize the hydrogen bonds induced by trehalose. They characterized the hydrogen bonding interactions in the hydrogel in its original hydrated state via attenuated total reflectance FTIR spectroscopy to understand how the hydrogel that they subjected to freeze-drying and grinding would not be the same as the original hydrated state. The results indicated the formation of intermolecular hydrogen bonds between trehalose and PAAm chains. The team then confirmed the covalent nature of such hydrogen-bonds, between trehalose and PAAm polymers using proton nuclear magnetic resonance. During additional experiments, Han et al. synthesized a range of hydrogels with 0, 10, 20, and 30 weight percent trehalose to PAAm polymer ratio, and characterized their mechanical properties by measuring stress-stretch curves of the notched and intact samples. Compared to pure PAAm hydrogels, the team noted significantly enhanced stretchability and strength of hydrogels soaked in solutions with different trehalose concentrations, while also increasing the shear modulus. The scientists also calculated the fracture toughness of the materials via pure shear tests.
Properties of modified materials
Han et al. next studied the temperature, dehydration properties and general applications of the materials. Since trehalose could impart anti-freezing properties to hydrogels, they explored the mechanical behaviors of trehalose-modified PAAm hydrogels at low temperatures. At minus 15 degrees Celsius, while trehalose-free PAAm hydrogels froze fully to become stiff and brittle, the trehalose-modified hydrogels retained their high stretchability and flexibility. The team measured the fracture toughness of the materials via pure shear tests, which increased with increasing trehalose content. In order to measure the transition temperature from a slurry to a frozen state, the team characterized the PAAm hydrogels with varying constituents via dynamic scanning calorimetry and showed how the transition temperature decreased with increased content of trehalose.
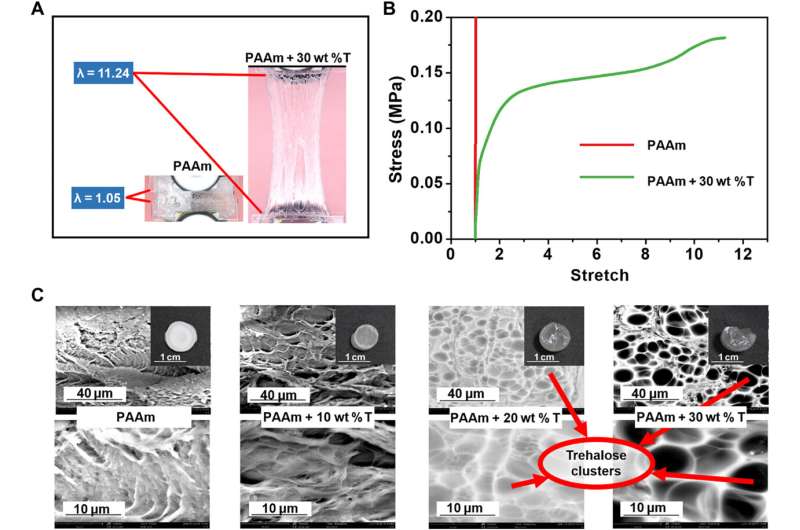
Applications and outlook
During the process of modified hydrogel preparation, the team included ammonium persulfate as an initiator to introduce free ions, including NH4+, S2O82−, HSO4−, H+, and SO42−, within the hydrogels to impart conductivity of about 0.32 S/m, which became non-conductive at low temperatures. The trehalose-modified materials maintained their conductivity in comparison at low temperatures due to their frozen nature. Using the modified materials, Han et al. illuminated a light-emitting-diode (LED) at minus 5 degrees Celsius, while materials without trehalose did not show similar capacity. The conductivities between 25 degrees Celsius and minus 15 degrees Celsius decreased, with increased mass fractions of trehalose, and the modified hydrogels also maintained good conductivity and excellent mechanical properties.
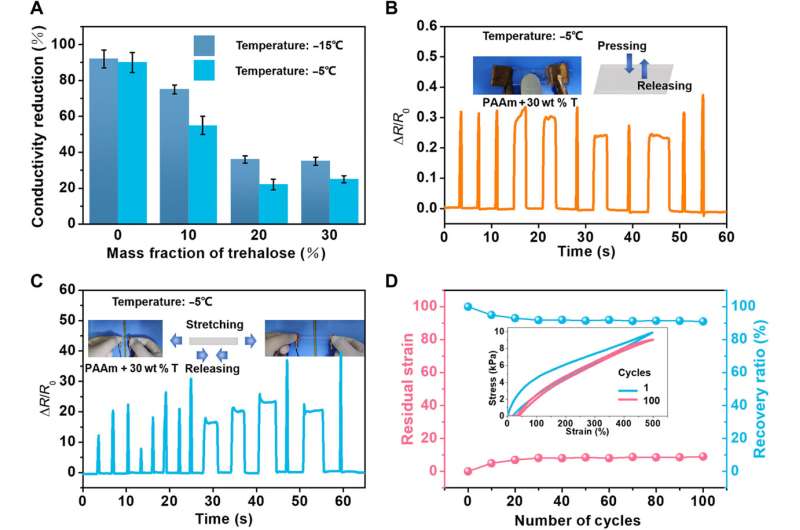
In this way, Zilong Han and colleagues developed a versatile strategy to increase the mechanical properties of various hydrogels, including PAAm (polyacrylamide), PVA (polyvinyl alcohol), and PAAm-alginate hydrogels. The modified materials maintained synthetic simplicity, while tolerating adverse environmental conditions. Trehalose functioned as the network-repairing agent by forming covalent-like hydrogen bonds between itself and the polymer chains. Consequently, with increasing trehalose content, the team noted significantly increased strength, stretchability and fracture toughness of the materials. The strategy delivers a versatile method to improve mechanical properties of hydrogels under a variety of conditions, to expand the scope of hydrogel applications.
A universal method to easily design tough and stretchable hydrogels
Zilong Han et al, A versatile hydrogel network–repairing strategy achieved by the covalent-like hydrogen bond interaction, Science Advances (2022). DOI: 10.1126/sciadv.abl5066
Jeong-Yun Sun et al, Highly stretchable and tough hydrogels, Nature (2012). DOI: 10.1038/nature11409
© 2022 Science X Network
Citation:
A versatile hydrogel network-repairing strategy (2022, March 15)
retrieved 16 March 2022
from https://phys.org/news/2022-03-versatile-hydrogel-network-repairing-strategy.html
This document is subject to copyright. Apart from any fair dealing for the purpose of private study or research, no
part may be reproduced without the written permission. The content is provided for information purposes only.
For all the latest Science News Click Here
For the latest news and updates, follow us on Google News.